Cutting DNA with restriction enzymes
Bacteria make enzymes called restriction endonucleases, or more commonly, restriction enzymes, that cut strands of DNA into smaller pieces. Bacteria use restriction enzymes to fight off attacking viruses, chopping up the viral DNA so that the virus can’t destroy the bacterial cell. Scientists use restriction enzymes in the lab to cut DNA into smaller pieces so that they can analyze and manipulate DNA more easily.Each restriction enzyme recognizes and cuts DNA at a specific sequence called a restriction site.
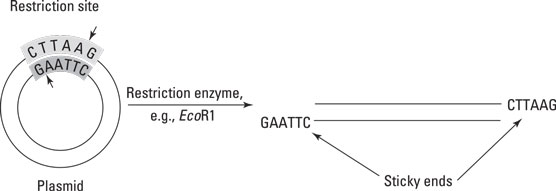
The restriction enzyme called EcoR1 cuts DNA at the sequence 5'GAATTC3'. If you mix DNA and a restriction enzyme, the enzyme will find all the restriction sites it recognizes and cut the DNA at those locations.
Restriction enzymes make cutting and combining pieces of DNA easy. For example, if you wanted to put a human gene into a bacterial plasmid, you’d follow these steps:
- Choose a restriction enzymes that forms sticky ends when it cuts DNA. Sticky ends are pieces of single-stranded DNA that are complementary and can form hydrogen bonds. Restriction enzymes that form sticky ends cut the DNA backbone asymmetrically so that a piece of single-stranded DNA hangs off each end. For example, the sticky ends shown in the figure have the sequences 5'AATT3' and 3'TTAA5'. A and T are complementary base pairs, so these ends could form hydrogen bonds and thus stick to each other.
- Cut the human DNA and bacterial plasmids with the restriction enzyme. If you cut a plasmid DNA and human DNA with the same restriction enzyme, all the DNA fragments will have the same sticky ends.
- Combine human DNA and bacterial plasmids. The two types of DNA have the same sticky ends, so some pieces of plasmid DNA and human DNA will stick together. Thus, some plasmids will end up with a human gene inserted into the plasmid.
- Use DNA ligase to seal the backbone of the DNA. DNA ligase will form covalent bonds at the cut sites in the DNA, sealing together any pieces of DNA that combined together.
cDNA with reverse transcriptase
Scientists use recombinant DNA technology to combine eukaryotic DNA with that of bacteria and then introduce eukaryotic genes into bacterial cells. However, bacteria can’t use eukaryotic genes to make proteins unless the introns are removed from the eukaryotic genes. Scientists get around this problem by creating intron-free eukaryotic genes in the form of complementary DNA (cDNA).cDNA is made from eukaryotic mRNA that has already been spliced to remove the introns.
The following figure shows the steps for making cDNA:- Isolate mRNA for the protein you’re interested in.
- Use the enzyme reverse transcriptase to make a single-stranded DNA molecule that is complementary to the mRNA. Reverse transcriptase is a viral enzyme that uses RNA as a template to make DNA.
- Use reverse transcriptase or DNA polymerase to make a partner strand for the DNA molecule, creating a finished double-stranded molecule of cDNA.
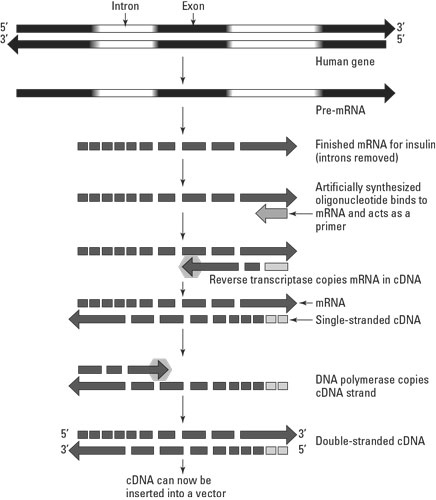
Cloning genes into a library
Scientists store the DNA they’re working with in DNA libraries, recombinant DNA molecules that contain the gene of interest. Once a gene is put into a DNA library, DNA cloning makes many identical copies of the gene. To clone a gene into a library, you first need to put the gene into a vector.A vector, such as a plasmid or virus, helps carry DNA into a cell.
The following figure illustrates the process for introducing a gene into a vector and then cloning the gene into a library.- Using the same restriction enzyme, cut the vector and the DNA containing the gene to be cloned. That way, the vector and the DNA to be cloned will have the same sticky ends.
- Mix the vector and DNA to be cloned together and add DNA ligase. Some vectors will pick up the genes to be cloned. DNA ligase will form covalent bonds, sealing the genes into the vectors. The vectors that pick up genes are recombinant.
- Introduce the vector into a population of cells. The vector will be reproduced inside the cells. Once the vector is reproduced, the gene has been cloned.
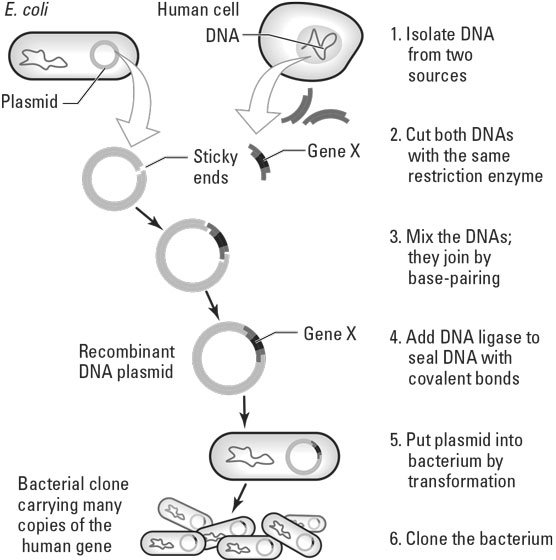
Cloning a gene isn’t the same thing as cloning an organism. Cloning a gene means making many copies of a gene, while cloning an organism means making an organism that is identical to another one (like the sheep Dolly). So, when someone talks about cloning, make sure that you know which version they mean!
DNA libraries are recombinant vectors that store genes and keep them handy for scientists.
DNA libraries make it easier for scientists to work with DNA they’re interested in, such as DNA from a particular type of cell or organism. Scientists use several types of DNA libraries:- DNA libraries contain fragments of DNA inserted into a vector.
- cDNA libraries contain fragments of cDNA inserted into vectors.
- Genomic libraries contain DNA fragments that represent the entire genome of an organism.
Finding a gene with DNA probes
After genes are cloned into a library, scientists use DNA probes to find the vectors that contain specific genes of interest. Probes are pieces of single-stranded DNA that are used to locate a particular DNA sequence (see the following figure).
Probes are made with a sequence that’s complementary to the sequence you’re looking for. Using a probe is like going fishing — you use the right bait (a complementary sequence) to catch something you want (a certain gene). Probes will attach with hydrogen bonds to their complementary sequence. For example, if you were looking for a gene that contained the sequence 5'TAGGCT3', you’d make a probe with the sequence 3'ATCCGA5'.Probes are also labeled with a fluorescent or radioactive marker so that you can locate them in a DNA sample. To use a probe to locate DNA, complete the following steps:
- Prepare a DNA sample to be probed for the gene of interest. You can look at DNA in many different forms — DNA in a gel, DNA attached to a microscope slide, and even DNA in colonies on a plate. To prepare any of these samples to be probed, you must treat the DNA with heat or chemicals to make it single-stranded and ready to pair with another strand of DNA.
- Wash your DNA probe over the surface of your DNA sample.
The probe will attach to its complementary sequence in the sample.
Nucleic acids probes.
- Locate your probe to find the gene of interest. A certain wavelength of light activates fluorescent probes. Radioactive probes are located by using the treated DNA to expose photographic film.